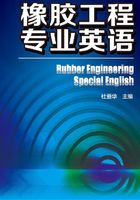
2.2 Polymer Characterization
2.2.1 Qualitative Analysis of Polymer
2.2.1.1 Fourier Transform Infrared Spectroscopy
Fourier transform infrared spectroscopy, a versatile and widely used analytical technique, relies on the creation of interference in a beam of light. FTIR spectrometer consists of an infrared source, an interference modulator(usually a scanning Michelson interferometer), a sample chamber, and an infrared detector. A source light beam is split into two parts and a continually varying phase difference is introduced into one of the two resultant beams. The two beams are recombined and the interference signal is measured and recorded as an interferogram. A Fourier transform of the interferogram provides the spectrum of the detected light. Computerised retrieval from data in a library of standard polymers has been used in the IR fingerprinting technique to facilitate polymer identification. Interference signals measured at the detector are usually amplified and then digitised. FTIR spectroscopy of butyl rubber(IIR) and maleic grafted butyl rubber(IIR-g-MAn) is shown in Figure 2.5.

Figure 2.5 FTIR spectroscopy of butyl rubber(ⅡR) and maleic grafted butyl rubber(ⅡR-g-MAn)
The principal reasons for choosing FTIR spectroscopy are that(ⅰ) the instruments record all wavelengths simultaneously and thus operate with maximum efficiency, and(ⅱ) Fourier transform infrared spectrometers have a more conventional optical geometry than do dispersive infrared instruments. These two factors lead to the following advantages.
Various methods have been used to prepare polymers for IR spectroscopy.
(1) Potassium bromide discs: the spectra were run either as potassium bromide discs(1.5~2μg polymer per 400mg potassium bromide) or as polymer films of varying thickness, up to 12μm, using a sodium chloride prism.
(2) Hot pressed film: Osland has described a heated press for the preparation of plastic films for analysis by IR spectroscopy. The press can produce films of reproducible thickness, as thick as 500μm.
Alternatively, the sample material can be dissolved in a suitable organic solvent and a film cast onto glass or a cell window.
(3)A new sample handling accessory with which films of constant thicknesses can be prepared has been introduced by phillips analytical. The plastic film press contains a thermostatically controlled oven unit that is calibrated up to 300℃. It also contains a cooling facility which may be connected to a low-pressure compressed air supply to cool the prepared films rapidly. Reproducible thickness is ensured by using a set of brass dies that can be heated and cooled quickly. The dies can produce films of 20μm, 50μm, 100μm, 200μm and 500μm thickness. Vinyl and carbonyl groups are the functions most likely to occur as the result of chain scission of polyethylene in air. Both are readily detectable by infrared spectroscopy, using the characteristic frequencies.
2.2.1.2 Raman Spectroscopy
Raman spectroscopy is similar to IR spectroscopy in that it investigates polymer structure focusing on the vibrational modes. Whereas IR is a result of energy being absorbed by a molecule from the ground state to an excited state.Raman spectroscopy is a scattering phenomenon where the energy of photons is much larger than the vibrational transition energies. Briefly, IR absorption occurs only if there is a change in the dipole moment during the vibration where as a change in polarizability is required for Roman scattering to occur.
Raman spectroscopy is an emission technique which involves irradiating a sample with a laser and collecting the scattered radiation. Most of the scattered radiation has the same wavelength as the laser. A very small fraction(approximately one millionth) of the scattered radiation is displaced from the laser wavelength by values corresponding to the vibrational frequencies of the sample. This is the Raman signal. The Raman spectroscopy information on molecular structure is complementary to that obtained from infrared spectroscopy. For example, some functional groups that give weak absorptions in the infrared, such as S—S,CC, and N
N, give strong Raman signals.
2.2.1.3 Nuclear Magnetic Resonance Spectroscopy
Nuclear magnetic resonance(NMR) spectroscopy is based on the measurement of absorption of electromagnetic radiation in the radio-frequency region of roughly 4 to 900MHz. In contrast to UV, visible, and IR absorption, nuclei of atoms rather than outer electrons are involved in the absorption process. Furthermore, to cause nuclei to develop the energy states required for absorption to occur, it is necessary to place the analyte in an intense magnetic field. In this chapter we describe the theory, instrumentation, and applications of NMR spectroscopy.
NMR spectroscopy is one of the most powerful tools available to chemicals and biochemists for elucidating the structure of chemical species. The technique is also useful for the quantitative determination of absorbing species.
1H and 13C NMR are vital tools for the characterization of polymeric materials. Solid-state NMR is frequently used to study such systems, but the brief discussion here will be confined to NMR in solution. 1H-NMR provides information relating to composition. This is particularly important for copolymers where such information may, for example, be used to determine reactivity ratios and, for vinyl polymers, can give an immediate indication of the presence of unreacted monomer. In some cases, for example poly(methyl methacrylate), the tacticity of the polymer can be readily established from the1H-NMR alone. However, it is often found that line widths in the 1H spectrum are relatively large compared with differences in chemical shift for different structural features. In such cases, details about tacticity may be obtained from the 13C-NMR spectrum. NMR is the powerful analytical technique used to establish the composition and microstructure of polymeric materials. Some specific chemical shift for certain methyl, methylene, and methine proton is listed in Table 2.1.
Table 2.1 Approximate chemical shifts for certain methyl,methylene,and methine protons

2.2.2 Quantitative Analysis of Polymer
With low molecular weight compounds, each compound has its own molecular weight: urea always has a molecular weight of 60.06, carbon dioxide always has a molecular weight of 44, and the molecular weight of anilin is always 93.12. A compound with a molecular weight of 100 can never be urea, and a compound with a molecular weight of 60 can never be anilin. However, with macromolecular compounds the situation is completely different. Thus, polystyrene can have a molecular weight of 80000, but it can also have a molecular weight of 5000, 800000, or even 8 million. Macromolecular substances do not have a certain definite and permanent molecular weight. Materials differ in their properties only by having a different molecular weight. For example, polystyrene with a molecular weight below 10000 is a brittle material without any mechanical strength, which can be easily rubbed into a fine powder, and which at a temperature of 50℃ becomes an oily liquid. On the other hand, molded polystyrene of a molecular weight of 250000, is a hard and strong glassy plastic, and polystyrene with a molecular weight over 1 million, is a compound which precipitates out of its solutions as a fibrous material and which at temperature over 100℃ turns into a tough rubbery material. Nearly all properties of macromolecular compounds change with the molecular weight, some only in a small region, up to a certain value of the molecular weight which is usually around 100000.However other properties, such as the viscosity, change continuously over the entire region of molecular weights obtainable. This is also why the plastics industry must be able to control the molecular weight of the products that are manufactured.
It is therefore very important in characterizing a polymeric material to give the molecular weight as a part of its description.Various of methods can be used to measure molecular weight, such as end group analysis, cryoscopic method, membrane osmosis, light scattering method, gel-permeation chromatography and viscosity method. Viscosity method and gel-permeation chromatography are the most commonly used methods.
2.2.2.1 Viscosity Method
The viscosity method is simple, convenient and accurate, and it is a common method. The calibration curve of intrinsic viscosity [η] versusMis known, determineMwsimply through viscosity measurements.
Measuring principle is direct correlation between polymer molecular weight and its solution viscosity, because the viscosity of the polymer solution is particularly larger than that of pure solvents. The solution viscosity is relative to the molecular weight of polymer and its size in the solvent due to the solvation effect. Molecular chains flow under higher friction because the length of molecular chain is greater than the solvent molecules, and molecular show the solvation effects. By means of viscosity measurement, the molecular weight, the size of the molecules in the solution and the solubility parameter of polymer materials could be characterized.
2.2.2.2 Gel-Permeation Chromatography
Gel-permeation chromatography(GPC) has become one of the most powerful and most frequently used techniques in the characterization of polymer molecular weight distribution. One of the reasons for this popularity is the ease(relative to other techniques such as ultracentrifugation, fractional precipitation, viscometry and other column fractionation methods) in obtaining a chromatogram which qualitatively represents the molecular weight distribution of polymers. The method is also relatively fast(2~3h) with minimum equipment set-up time. It requires no more effort than dissolving, filtering and injecting the sample into the chromatograph to start the analysis. Gel permeation chromatography(GPC) is also an efficient analysis method of separation or purification, especially for the macromolecules with different molecular weights(MWs). The quantitative ability as presented by GPC has been employed accurately and effectively in rubber material research areas.
2.2.2.3 Polydispersity
The ratio ofMw/Mnis a measure of polydispersity and is called the polydispersity index. If one is less interested in the course of the distribution curve than in the width of the distribution, then one can be satisfied with two molecular weight determinations, i.e., an osmotic determination giving the averageMnand a sedimentation or light-scattering measurement which gives essentially anMwvalue. The ratioMw/Mn=1 means that the product consists of completely homogeneous macromolecules(sometimes one finds the valueMw/Mn-1 defined as the inhomogeneity). The further the ratioMw/Mndiffers from 1, the less homogeneous the product is. For living polymerization polymer,Mw/Mnlies between 1.05 and 1.2. On the other hand for a technical bulk-polymer,Mw/Mnmay be approximately 10. For polymers which have been polymerized by radical initiation at constant temperature and not to very high conversion(less than 80%), the ratioMw/Mnlies between 2 and 3(with normal distributionMw/Mnis 1.5 and 2, respectively).
2.2.3 Thermal Analysis
Major instrumentation involved with the generation of thermal property behavior of materials includes thermogravimetric analysis(TGA), differential scanning calorimetry(DSC), differential thermal analysis(DTA). There are several different modes of thermal analysis described as DSC. DSC is a technique of nonequilibrium calorimetry in which the heat flow into or away from the polymer is measured as a function of temperature or time. This is different from DTA where the temperature difference between a reference and a sample is measured as a function of temperature or time. Presently available DSC equipment measures the heat flow by maintaining a thermal balance between the reference and sample by changing a current passing through the heaters under the two chambers. For instance, the heating of a sample and reference proceeds at a predetermined rate until heat is emitted or consumed by the sample. If an endothermic occurrence takes place, the temperature of the sample will be less than that of the reference. The circuitry is programmed to give a constant temperature for both the reference and the sample compartments. Excess current is fed into the sample compartment to raise the temperature to that of the reference. The current necessary to maintain a constant temperature between the sample and reference is recorded. The area under the resulting curve is a direct measure of the heat of transition.
The advantages of DSC and DTA over a good adiabatic calorimeter include speed, low cost, and the ability to use small samples. Sample size can range from 0.5 mg to 10g. A resultant plot ofTas a function of time or temperature is known as a thermogram. Since the temperature difference is indirectly proportional to the heat capacity, the curves resemble inverted specific heat curves.
Possible determinations from DSC and DTA measurements include the following:heat of transition,heat of reaction,sample purity,phase diagram,specific heat,sample identification,percentage incorporation of a substance,reaction rate,rate of crystallization or melting,solvent retention,activation energy,the glass transition temperature(Tg). Thus, thermo calorimetric analysis can be a quite useful tool in describing the chemical and physical relationship of a polymer with respect to temperature.
In TGA, a sensitive balance is used to follow the weight change of a polymer as a function of time or temperature. Usual sample sizes for commercial instruments are in the range of 0.1mg to 10g with heating rates of 0.1℃/min to 50℃/min. The most commonly employed heating rates are 10℃/min, 15℃/min, 20℃/min, 25℃/min, and 30℃/min. In making both TGA and thermo calorimetric measurements, the same heating rate and flow of gas should be employed to give the most comparable thermograms. TGA can be used to determine the following:sample purity,identification,solvent retention,reaction rate,activation energy, and heat of reaction.
Words and expressions
propagating chain:增长链
aqueous emulsion:水乳液
heterogeneous[,hɛtərə'dʒiniəs, -'dʒinjəs]:多相的,不均匀的
undiluted[,ʌndaɪ'luːtɪd]:未稀释的
droplet['draːplət]:液滴
micelle[maɪ'sel]:胶束
electrostatic repulsion:排斥
trace amounts:微量
impuritie[ɪm'pjʊərɪtɪs]:杂质
vinyl ether:乙烯醚
para-substituted:对位取代
gegenion['geɪgənaɪən]:反离子,抗衡离子
stereoregular[stɪərɪr'regjʊlə]:有规立构的
isotactic[aɪsoʊ'tæktɪk]:全同立构的
syndiotactic[sɪndɪoʊ'tæktɪk]:间同立构的
alkali['ælkə,laɪ]:碱
contaminant[kən'tæmənənt]:污染物,致污物
telechelic polymer:遥爪聚合物
titanium tetrachloride[taɪ'teniəm ,tɛtrə'klɔr,aɪd]:四氯化钛
aluminium[,æljə'mɪniəm]:铝,铝的
atactic[eɪ'tæktɪk]:无规立构的
interference[,ɪntər'fɪrəns]:干涉,干扰
spectrometer[spek'traːmɪtə(r)]:分光计
potassium[pə'tæsiəm]:钾
sodium['soʊdiəm]:钠
thermostatically [,θɝmə'stætɪkli]:恒温地;自动调节温度地
calibrate['kælə,bret]:校准,使标准化
vibrational[vɑɪ'breɪʃənəl]:振动的,摇摆的
photon['foʊtaːn]:光子,光量子
dipole moment:偶极矩
emission[ɪ'mɪʃən]:辐射,排放
scattered radiation:散射辐射
radio-frequency:射频,无线电频率
methine['meθiːn]:次甲基
anilin[æ'nəlɪn]:苯胺
urea[jʊ'riːə]:尿素,脲
brittle['brɪtl]:易碎的,脆的
cryoscopic method:冰点降低法
membrane['mɛm,bren]:隔膜,薄膜
osmosis[ɑːz'moʊsɪs]:渗透(作用)
chromatography[,kroʊmə'taːgrəfi]:色谱分析法
intrinsic viscosity:特性黏度
ultracentrifugation [ʌltrəsentrɪfjʊ'ɡeɪʃn]:超速离心法
fractional precipitation:分级沉淀,分段沉淀
purification[,pjʊrəfɪ'keʃən]:提纯,洗净
sedimentation[,sɛdəmən'teʃən, -mɛn-]:沉淀,沉降
nonequilibrium['nɒnɪkwɪ'lɪbrɪrm]:不平衡的;非平衡态
calorimetry[,kælə'rɪmɪtriː]:热量测定
circuitry['sɜːrkɪtri]:电路,电路图
adiabatic[,ædɪr'bætɪk]:绝热的
thermogram['θɜːmə,græm]:温度记录图