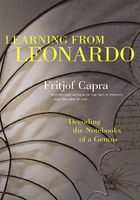

1
The Movements of Water
Among the four classical elements, water held by far the greatest fascination for Leonardo. Throughout his life, he studied its movements and flows, drew and analyzed its waves and vortices, and speculated about its role as the fundamental “vehicle of nature” (vetturale della natura) in the macrocosm of the living Earth and the microcosm of the human body.
Leonardo’s notes and drawings about his observations and ideas on the movement of water fill several hundred pages in his Notebooks. They include elaborate conceptual schemes and portions of treatises in the Codex Leicester and in Manuscripts F and H, as well as countless drawings and notes scattered throughout the Codex Atlanticus, the Codex Arundel, the Windsor Collection, the Codices Madrid, and Manuscripts A, E, G, I, K, and L. The sheer bulk of Leonardo’s writings on water duly impressed his contemporaries and succeeding generations of historians. In fact, water was the only subject, apart from painting, of which an extensive compilation of handwritten transcriptions from the Notebooks was made. This collection of notes, transcribed in the seventeenth century and comprising 230 folios, was published in 1828 in Bologna under the title Della natura, peso, e moto dell’acque (On the Nature, Weight, and Movement of Water).
Carrier and Matrix of Life
Leonardo was fascinated by the nature and movements of water for several reasons. I believe that, ultimately, they all have to do with his persistent quest to understand the nature of life, which informed both his science and his art. Leonardo’s science is a science of living, organic forms, and he clearly recognized that all organic forms are sustained and nourished by water:
PRECEDING A stream running through a rocky ravine, c. 1483 (detail, see fig. 2-5).
FACING “Water falling upon water,” c. 1508–9 (see fig. 1-13).
It is the expansion and humor of all living bodies.
Without it nothing retains its original form.
The term “humor” is used here in its medieval sense of a nourishing bodily fluid. In another Notebook, Leonardo wrote: “[Water] moves the humors of all kinds of living bodies.” Being a painter, he had ample experience with water as a solvent and accurately described this chemical property: “It has nothing of itself, but moves and takes everything, as is clearly shown when distilled.”
Leonardo’s view of the essential role of water in biological life is fully borne out by modern science. Today we know not only that all living organisms need water for transporting nutrients to their tissues but also that life on Earth began in water. The first living cells originated in the primeval oceans more than three billion years ago, and ever since that time all the cells that compose living organisms have continued to flourish and evolve in watery environments. Leonardo was completely correct in viewing water as the carrier and matrix of life.
One of the fundamental principles of Leonardo’s science is the similarity of patterns and processes in the macro- and microcosm. Accordingly, he compared the “water veins” of the Earth to the blood vessels of the human body (see p. 26). As blood nourishes the tissues of the body, so water nourishes the Earth’s vegetation with its “life-giving moisture.”
And as water expands when it vaporizes in the heat of the sun and “becomes mingled with the air,” so blood by its warmth spreads into the periphery of the body.
Indeed, we shall see that Leonardo described in great detail how blood carries nutrients to the bodily tissues and that he developed an ingenious, though incorrect, theory of how body heat is generated by the turbulent flows of blood in the chambers of the heart (see p. 296).
In his paintings, Leonardo represented water as the carrier of life not only in the scientific sense but also symbolically, in the religious sense. According to the Christian theology that shaped the culture in which he lived, the faithful receive a new spiritual life in the sacrament of baptism, and water is the medium that conveys this sacrament. In the words of the Bible, baptism is rebirth of water and spirit (John 3:5). Several of Leonardo’s paintings contain variations on this fundamental religious theme, often integrating the religious symbolism with his scientific understanding of the life-giving quality of water.
This integration is already apparent in the very first record we have of Leonardo as a painter, when he was still an apprentice in the workshop of Andrea del Verrocchio in Florence. Around 1473, when Leonardo was twenty-one, Verrocchio let the youth paint one of two angels and parts of the background in his picture of the Baptism of Christ (plate 2). Leonardo painted a wide, romantic stretch of hills and pinnacles of rocks of the kind that would form the backgrounds in many of his later paintings, and to that he added a long watercourse, flowing from a pool in the far distance all the way to the foreground, where it forms small waves rippling around the legs of Christ. While these ripples in the foreground represent the lifegiving water of the sacrament, the watercourse in the background, cutting through arid rocks and flowing into a fertile valley, portrays water as the carrier of biological life in the macrocosm of the Earth.
This theme is expanded and elaborated in several of Leonardo’s later paintings, in particular, in three of his masterpieces—the Virgin of the Rocks (plate 8), the Mona Lisa (plate 11), and the Madonna and Child with Saint Anne (plate 7). In the Virgin of the Rocks, Leonardo depicts a prophetic meeting of the infant Christ with the infant Saint John long before the Baptism. According to a fourteenth-century legend, this meeting took place during the Holy Family’s flight into Egypt, where they lived in the wilderness after their escape from Herod’s massacre of the innocents. Leonardo has placed the scene in front of a rocky grotto and turned it into a complex meditation on the destiny of Christ, expressed through the gestures and relative positions of the four protagonists, as well as in the intricate symbolism of the surrounding rocks and vegetation. An angel conspicuously points to the Baptist, directing our attention to his spiritual dialogue with Christ, while Mary tenderly protects the children with her outstretched arms.
As in Verrocchio’s Baptism, a mountain stream emerges in the far distance from the misty atmosphere surrounding pinnacles of rocks and breaks through the rocky landscape, flowing all the way to the foreground of the painting where it runs through a small pool—an allusion to the Baptism. However, the rocks are rendered here in much more detail and with astonishing geological accuracy (see pp. 77ff.), and the luxuriant vegetation in the grotto’s moist environment is clear testimony to the generative powers of water, presented by the artist in a subtle synthesis of scientific knowledge and religious symbolism (see pp. 102ff.).
The Mona Lisa is Leonardo’s deepest meditation on the mystery of the origin of life—the theme that was foremost in his mind during his old age. The central theme of the artist’s most famous painting is life’s procreative power, both in the female body and in the body of the living Earth. Essential to this power is the fundamental role of water as the life-giving element (see pp. 318ff.).
The theme of the origin of life is taken up again in the Saint Anne, which Leonardo painted around the same time as the Mona Lisa. Here the artist returned once more to exploring the mystery of life within a religious context. The painting shows Mary, her mother Saint Anne, and the Christ child together with a lamb in a highly original composition. Its theological message can be viewed as a continuation of Leonardo’s long meditation on the destiny of Christ, which began with the Virgin of the Rocks.
Once more, the familiar mountain lakes and jagged rocks rise high into the background, although they are less imposing than those behind the Mona Lisa. In both paintings, the central theme is the mystery of the origin of life in the human body and in the body of the Earth. In the Saint Anne, this is rendered even more complex by the presence of three generations and by the myth of the virgin birth. There is a double mystery here: the immaculate conception of Mary by Saint Anne and that of Christ by Mary. To emphasize the analogy between human nature and the Earth, Leonardo has mirrored the three generations in the painting’s foreground by three tiers of mountain lakes, interlinked by small waterfalls, in the background.
What these four paintings—the Baptism, the Virgin of the Rocks, the Mona Lisa, and the Saint Anne—have in common is Leonardo’s extended reflection on water as the life-giving element in the macrocosm of the Earth and the microcosm of human existence. Drawing on his scientific understanding, his artistic genius, and his great familiarity with religious symbolism, Leonardo expressed this meditation in a series of masterpieces that have become enduring icons of European art.
Nature’s Fluid Forms
Another reason Leonardo was so fascinated by water is that he associated it with the fluid and dynamic nature of organic forms. Ever since antiquity, philosophers and scientists had recognized that biological form is more than shape, more than a static configuration of components in a whole. There is a continual flux of matter through a living organism, while its form is maintained; there is growth and decay, regeneration and development. This dynamic conception of living nature is one of the main themes in Leonardo’s science and art. He portrayed nature’s forms—in mountains, rivers, plants, and the human body—in ceaseless movement and transformation. And, knowing that all organic forms are sustained by water, he sensed a deep connection between their fluidity and the fluidity of water.
As Leonardo observed the flow of the life-giving element, he marveled at its endless versatility and adaptability. “Running water has within itself an infinite number of movements,” he noted in Manuscript G, “sometimes swift, sometimes slow, and sometimes turning to the right and sometimes to the left, now upwards and now downwards, turning over and back on itself, now in one direction and now in another, obeying all the forces that move it.” In the Codex Atlanticus he wrote: “Thus, joined to itself, water turns in a continual revolution. Rushing this and that way, up and down, it never rests, neither in its course nor in its nature. It owns nothing but seizes everything, taking on as many different characters as the places it crosses.”
In addition, Leonardo carefully studied the actions of water in the erosion of rocks and river banks, its transformations into solid and gaseous forms (known in science today as phase transitions), and its properties as a chemical solvent. He never divided these diverse properties into separate categories but saw them all as different aspects of the fundamental role of water in nourishing and sustaining life:
Without any rest, it is ever removing and consuming whatever borders upon it. So at times it is turbulent and goes raging in fury, at times clear and tranquil it meanders playfully with gentle course among the fresh pastures. At times it falls from the sky in rain, snow, or hail. At times it forms great clouds out of fine mist. At times it moves of itself, at times by the force of others. At times it increases the things that are born with its life-giving moisture. At times it shows itself either fetid or full of pleasant odors. Without it nothing can exist among us.
For Leonardo, the fluid and ever-changing forms of water were extreme manifestations of the fluidity that he saw as a fundamental characteristic of all the forms of nature. He also noticed, however, that certain flows of water can produce forms that are surprisingly stable: eddies, vortices, and other forms of turbulence known to scientists today as coherent structures (see p. 55). He observed and sketched a great variety of these relatively stable turbulent structures, and I believe that his lifelong fascination with them came from his deep intuition that, somehow, they embodied an essential characteristic of living, organic forms.
Today, from our modern perspective of complexity theory and the theory of living systems, we can say that Leonardo’s intuition was absolutely correct. The fundamental characteristic of a water vortex—for example, the whirlpool that is formed as water drains from a bathtub—is that it combines stability and change. The vortex has water continuously flowing through it, and yet its characteristic shape, the well-known spirals and narrowing funnel, remains remarkably stable. This coexistence of stability and change is also characteristic of all living systems, as complexity and systems theorists recognized in the twentieth century.
The process of metabolism, the hallmark of biological life, involves a continual flow of energy and matter through a living organism—the intake and digestion of nutrients and the excretion of waste products—while its form is maintained. Thus, metaphorically, one could visualize a living organism as a whirlpool, even though the metabolic processes at work are not mechanical but chemical.
Leonardo never used the analogy between the dynamic of a water vortex and that of biological metabolism, at least not in the Notebooks that have come down to us. However, he was well aware of the nature of metabolic processes. Indeed, we shall see that his detailed description of tissue metabolism in connection with the flow of blood in the human body must be seen as one of his most astonishing scientific insights (see p. 312). Thus, it seems not too far-fetched to assume that he was so fascinated by whirlpools and vortices because he intuitively recognized them as symbols of life—stable and yet continually changing.
A Source of Power
Leonardo saw water not only as the life-giving element but also as the principal force shaping the Earth’s surface and as a major source of power, which could be harnessed by human ingenuity. In his time, three hundred years before the Industrial Revolution, the windmill, the water wheel, and the muscles of beasts provided the only power to drive human technologies, and among those Leonardo thought that water had the greatest potential. At the age of fifty, when he was famous as a painter throughout Europe and known as one of Italy’s leading military and hydraulic engineers, he dreamed of a grand scheme for a kind of “industrial” canal along the river Arno between Florence and Pisa. He imagined that such a waterway would provide irrigation for the surrounding fields as well as energy for numerous mills that could produce silk and paper, drive potters’ wheels, saw wood, forge iron, burnish arms, and sharpen metal.
Leonardo’s ambitious project was never realized, but it was a prophetic vision. Centuries later, the powers of steam and hydroelectricity would indeed transform human civilization.
As an engineer, Leonardo was also well aware of the destructive power of water. In the plains of northern Italy, at the foot of the Alps, an elaborate system of canals had been built for irrigation and for commercial navigation, and one of the main challenges faced by hydraulic engineers was how to protect these canals from the flooding of their tributaries (see p. 32). This flooding happened periodically during heavy autumn rains and after a sudden spring melting of the Alpine snows. Leonardo paid great attention to these inundations, which could be very violent. He had witnessed a catastrophic flooding of the Arno in his native Tuscany at the age of fourteen. This childhood experience must have left a deep impression on him and perhaps was the cause of his morbid fascination with floods, which he considered the most frightening of all cataclysmic events. “How can I find words to describe these abominable and frightening evils, against which there is no human defense?” he wrote in the Codex Atlanticus. “With swollen waves rising up, it devastates high mountains, destroys the strongest embankments, and tears out deeply rooted trees. And with voracious waves, laden with the mud of plowed fields, it carries off the fruits of the hard work of the miserable and tired tillers of the soil, leaving the valleys bare and naked with the poverty it leaves in its wake.”
As a hydraulic engineer, Leonardo invented special machines for digging canals, improved the existing systems of locks, drained marshes, and modified the flows of rivers to prevent damage to properties along their banks. As an architect, he designed elaborate landscape gardens with splendid fountains, running water for cooling wine, sprinkler systems for refreshing guests during the hot summers, and automatic musical instruments played by water mills.
He decided early on that his reputation and skills in hydraulic engineering and landscape design would be grounded in a thorough understanding of the flow of water. In his science and his art, Leonardo never tired of observing, analyzing, drawing, painting, and studying how water moves through the air, the blood vessels of the human body, the vascular tissues of plants, and the seas and rivers of the living Earth.
The Water Cycle
Since Leonardo’s science was based on repeated observations of natural phenomena combined with meticulous analysis, it is not surprising that he had an accurate understanding of the evaporation and condensation of water and was able to describe it clearly. “Readily it rises up as vapors and mists,” he wrote in Manuscript A, “and, converted into clouds, it falls back as rain because the minute parts of the cloud fasten together and form drops.”
A slightly more detailed description can be found in the Codex Arundel:
At times it is bathed in the hot element and, dissolving into vapor, becomes mingled with the air; and drawn upward by the heat, it rises until, having found the cold region, it is pressed closer together by its contrary nature, and the minute particles become attached together.
He was also well aware of the fact that water continually cycles through the earth and atmosphere: “We may conclude that the water goes from the rivers to the sea, and from the sea to the rivers, thus constantly circulating and returning.” Taken together, these statements seem to indicate that Leonardo had a clear understanding of the essential phases of the water cycle—how water in the oceans, heated by the sun, evaporates into the air; how it rises into the atmosphere until cooler temperatures cause it to condense into clouds; how minute particles in the clouds coalesce into larger drops that precipitate as rain or snow; and how this precipitation eventually flows into rivers that carry it back into the oceans.
In actual fact, however, Leonardo’s views of the water cycle were far from clear. He considered several different explanations, struggled for many years because none of them satisfied his critical mind, and arrived at the correct view only in his old age, in his early sixties. How are we to understand that? What prevented a man of his genius from understanding a natural process that seems so evident to us today?
The answer to the puzzle provides a fascinating example of the tremendous power of the philosophical framework known today as a scientific paradigm—the constellation of concepts, values, and perceptions that form the intellectual context of all scientific investigations. One of the foundations of the medieval worldview was the conviction that nature as a whole was alive, and that the patterns and processes in the macrocosm were similar to those in the microcosm. This analogy between macro- and microcosm, and in particular between the Earth and the human body, goes back to Plato and had the authority of common knowledge in the Middle Ages and the Renaissance.
Leonardo fully embraced it as one of the guiding principles of his science and discussed it repeatedly (see pp. 65ff.). Whenever he explored the forms of nature in the macrocosm, he also looked for similarities of patterns and processes in the human body, and so it was natural for him to compare the “water veins” of the Earth to the blood vessels of the body.
Our modern systemic conception of life fully validates Leonardo’s method of exploring similarities between patterns and processes in different living systems, and his view of the Earth as being alive has reappeared in today’s science, where it is known as Gaia theory. However, Leonardo ran into difficulties with his comparisons between the living Earth and the living human body because he extended them beyond the similarity of patterns to comparisons of forces and material structures. One of the important insights of modern systems and complexity theories has been that, even though patterns of relationships between the components and processes of two different living systems may be similar, the processes themselves and the forces and structures involved in them may be quite different.
It took Leonardo the better part of his life to realize this, but he clearly did so in his old age.
Since the total amount of water on Earth is finite, Leonardo argued, the water carried into the sea by the rivers must somehow cycle back to their sources, “thus constantly circulating and returning.” Since he conceived of water as a “humor” that nourishes the Earth just as the blood nourishes the human body, he imagined that there must be water veins inside the body of the living Earth corresponding to the blood vessels in the bodies of animals and humans:
The body of the Earth, like the bodies of animals, is interwoven with a network of veins which are all joined together, and are formed for the nutrition and vivification of that Earth and of its creatures. They originate in the depths of the sea, and there after many revolutions they have to return through the rivers, created high up by the bursting of these veins.
This was the traditional view, put forward by philosophers from Aristotle to the Renaissance: inside the living Earth, there is a system of water veins, in which the water circulates like the blood in a living body, until the veins eventually break in the high mountains. There, the water emerges from mountain springs, is collected by the rivers, and flows back into the sea. Leonardo realized, of course, that rivers are also fed by rainwater and melting snow. But for many years he maintained that their principal sources were the internal veins of the Earth. Even though he encountered many logical inconsistencies, he was unwilling for the longest time to abandon the powerful analogy between the circulation of water in the Earth and that of blood in the human body. “The water that rises within the mountains,” he wrote in his early forties, “is the blood that keeps these mountains alive.”
Leonardo’s scientific mind was not content with the beautiful metaphorical description of water as “the blood that keeps the mountains alive.” He needed to explain how the water actually rises up to the mountain springs through internal channels. It was clear to him that some forces counteracting gravity had to be at work:
The water which sees the air through the broken veins of the high mountain summits is suddenly abandoned by the power which brought it there, and when it escapes from these forces that elevated it to the summit, it freely resumes its natural course.
But what exactly were these forces? To find an answer, Leonardo used a method that was characteristic of all his investigations. Understanding a phenomenon, for him, always meant connecting it with other phenomena through a similarity of patterns. In this case, he identified two similar phenomena—how the blood in the human body rises to the head and how the sap in a plant rises up from its roots—and he assumed that the same forces were acting in all three examples:
The same cause which moves the humors in all kinds of living bodies against the natural course of gravity also propels the water through the veins of the Earth, wherein it is enclosed, and distributes it through small passages. As the blood rises from below and pours out through the broken veins of the forehead, and as the water rises from the lower part of the vine to the branches that are cut, so from the lowest depth of the sea the water rises to the summits of the mountains where, finding the veins broken, it pours down and returns to the low-lying sea.
Having established this similarity of patterns, Leonardo then set out to identify the common forces underlying them. Over the years, he tried and then rejected several explanations. At first, he thought that the water was drawn up inside the mountains as steam by the heat of the sun, and he suggested that this process was similar to blood rising to a man’s head when it is hot:
When the sun warms a man’s head, the blood increases and rises so much with other humors that, by pressuring the veins, it often causes headaches.
“The heat of fire and sun by day,” Leonardo argued, “have the power to extract the moisture from the low places of the mountains and draw it up high in the same way as it draws the clouds and extracts their moisture from the bed of the sea.”
Subsequently, however, he discovered two reasons why this explanation could not work. He noted that on the highest mountain tops, closest to the heating sun, the water remains cold and is often icy. Moreover, with this mechanism the greatest amount of water should be drawn up in summer when the sun is hottest, but mountain rivers are often lowest at this time.
In a second explanation, Leonardo suggested that the water might be drawn up in a process of distillation, fueled by the Earth’s internal heat. He was aware of the presence of fire within the Earth from observations of hot springs and volcanoes, and he had also experimented with several types of distillation apparatus. Perhaps, he suggested, the interior fires of the Earth boil water in special caverns until it rises as vapor to the roofs of those caverns, “where, coming upon the cold, it suddenly changes back into water, as one sees happen in a retort, and goes falling down again and forming the beginnings of rivers.”
Again, Leonardo found an argument against his own explanation. Such extensive distillation, he realized, would keep the roofs of internal caverns wet from the rising steam, but he remembered from his explorations of mountain caves that they were often bone dry.
A third proposal was based on the observation that water rises in a vacuum within an enclosed space. Leonardo was quite familiar with this phenomenon. One of his early inventions, when he was still working in Verrocchio’s bottega, had been a method of creating a vacuum to raise water by means of a fire burning in a closed bucket. Now he hypothesized that the internal fires might rarefy the air in the Earth’s caverns and thus raise the water to the top. However, he soon realized that this would not work, because additional air would enter the cavern through the openings of the mountain springs and would thus stop the siphoning action of the vacuum.
On a folio of the Codex Leicester, Leonardo summarized both the distillation model and the siphoning model together with their counterarguments. He illustrated the discussion with a drawing showing the cross section of a mountain with interior veins running from the sea all the way up to the top where they connect with two large caverns. Right below, we see clear sketches of the two processes of distillation and siphoning (fig. 1-1). An accompanying folio contains numerous drawings illustrating experiments with various siphons.

FIG. 1-1. Models of water circulation by distillation and by siphoning action. Codex Leicester, folio 3v (detail).
As yet another alternative, Leonardo suggested that the water might be drawn up inside the mountains by some process similar to the action of a sponge, but that vague idea did not satisfy him either. “If you should say that the Earth’s action is like that of a sponge,” he countered, “the answer is that, even if the water rises to the top of that sponge by itself, it cannot then pour down any part of itself from this top, unless it is squeezed by something else, whereas with the summits of the mountains one sees the opposite, for there the water always flows away by itself without being squeezed by anything.”
After many years of considering various explanations and finding counterarguments to all of them, Leonardo finally realized that his analogy between the blood vessels of the human body and the water veins of the Earth was too narrow; that in the water cycle, the water does not circulate inside the mountains but rises as vapor through the air, drawn up by the heat of the sun, and then falls as rain on the mountaintops. On a folio of the Windsor Collection, written after 1510 when he was around sixty, Leonardo stated unequivocally that “the origin of the sea is contrary to the origin of the blood,” because the rivers “are caused entirely by the aqueous vapors raised up into the air.”
Around the same time, in a note in Manuscript G about water as the carrier of minerals, Leonardo stated quite casually, as a matter of fact, that the rivers are produced by clouds:
The saltiness of the sea is due to the numerous water veins, which in penetrating the earth find the salt mines, and dissolving parts of these carry them away with them to the ocean and to the other seas from whence the clouds, originators of the rivers, never raise them up.
From our contemporary perspective, Leonardo’s long intellectual struggle to understand the water cycle is extremely interesting. His successive theoretical formulations are quite similar to the theoretical models that are characteristic of modern science. Like scientists today, he continually tested his models and was ready to replace them when he found that they contradicted some empirical evidence. Moreover, as he progressed, he kept in mind the analogies and interconnecting patterns to phenomena in other areas, and revised his theories about those other phenomena accordingly. Thus, as he modified his explanations of the water cycle, he also modified similar models of the functioning of the heart and the flow of blood in the human body (see pp. 284–85).
In the end, Leonardo came to realize that, although water and blood both carry nutrients to living systems (as we would say today) and both cycle continually, the pathways of the two cycles and the forces driving them are quite different. During the years of 1510–15, when he finally reached a clear understanding of the water cycle, he also came to the conclusion that the blood in the human body is moved by the pumping action of the heart (see pp. 290ff.). That Leonardo was able, in his old age, to abandon the narrow analogy between the circulation of blood in the human body and the circulation of water in the body of the Earth, which had been firmly established in medieval philosophical thought, is an impressive testimony to his intellectual integrity, his perseverance, and the power of his scientific method.
When he was in his early sixties and reached his full understanding of the water cycle and the movement of blood in the human body, Leonardo also produced his most sophisticated writings in botany, in which he described the transport of “vital sap” through the vascular tissues of plants (see pp. 120ff.). It would be fascinating to know how his insights into the circulation of water and blood affected his ideas of how water rises through the plant tissues from the roots to the top. Today we know that this is a consequence of the evaporation of water from the leaves and of its intermolecular forces—the “cohesion in itself,” as Leonardo called it (see p. 41). Unfortunately, we are not likely to ever know Leonardo’s last thoughts on these matters since the manuscript that may have contained his definitive treatise on botany has been lost.
From Hydraulic Engineering to the Scientific Study of Flow
The majority of Leonardo’s extensive collections of notes and drawings on the flow of water were concerned with problems of hydraulic engineering and with the phenomenon of flow itself. In the Renaissance, the latter was a subject unique to Leonardo. The movement of rigid bodies had been studied since antiquity. In contrast, although hydraulic engineers had produced magnificent works—from the great aqueducts and luxurious thermal baths of the Romans to the ingenious navigation locks of the early fifteenth century—it had not occurred to any of them to wonder how flowing water could be described mathematically. Nor did they attempt to explore the fundamental laws of fluid flow, the subject of our modern discipline of fluid dynamics. Leonardo did both. His investigations, drawings, and attempted mathematical descriptions of flow patterns in water and air must be ranked among his most original scientific contributions, leading him to discoveries that would reappear only centuries later.
When he was first employed as painter and “ducal engineer” at the Sforza court in Milan in 1490, Leonardo had already spent eight years in the capital of Lombardy, which was a vibrant trading center of tremendous wealth and a major seat of political power in northern Italy. During those years, he had not only painted the Virgin of the Rocks and a highly original portrait of the mistress of Ludovico Sforza, but had also undertaken an extensive program of self-education during which he systematically studied the principal fields of knowledge of the time.
From his first years in Lombardy, Leonardo was fascinated by the engineering problems involved in the region’s elaborate system of canals. During the previous three centuries, hydraulic engineering in northern Italy had reached a level of considerable sophistication. The wealth of the Lombard region was dependent on the control of water and on land reclamation from marshes. Hydraulic engineering was needed to reduce damage from the periodic flooding of Alpine rivers, to supply the cities with water, to keep ports working, for irrigation, and for commercial navigation. The great canals of Lombardy, wide enough to let two large barges pass, interconnected the principal rivers of the area and featured a series of sophisticated locks for overcoming differences of water levels.
As ducal engineer at the Sforza court, Leonardo was probably in charge of all hydraulic works and thus became thoroughly familiar with the existing technologies and the problems that needed to be solved. Indeed, the Codex Leicester contains a vast number of observations on practical hydraulic problems in rivers and canals. Before Leonardo, such knowledge had been transmitted mostly orally, and the approach of the Lombard engineers was purely empirical: all their practices and rules were based on the success or failure of previous similar works. This did not satisfy Leonardo’s scientific mind. He needed to know the reasons behind the empirical rules, and so he embarked on his lifelong studies of the laws of fluid flow, beginning with the basic dynamics of the flow of rivers and proceeding to complex patterns of turbulent flow.
Even in the midst of his theoretical studies, Leonardo always kept their practical applications in mind. For example, during a discussion of the flow of water around immersed obstacles, he noted: “The science of these objects is of great usefulness, for it teaches how to bend rivers and avoid the ruins of the places struck by them.” In Manuscript F, written during the same period as the Codex Leicester, we find the following admonition: “When you put together the science of the movements of water, remember to put beneath each proposition its applications, so that such science may not be without its uses.”
In Leonardo’s time, the scientific study of flow phenomena, now known as fluid dynamics, was entirely new. It was a field of study he himself created single-handedly. However, in view of his dynamic conception of the world and his practice of portraying nature’s forms in his drawings and paintings as being in ceaseless movement and transformation, such a study must have seemed completely natural to him. Indeed, flow was one of the dominant themes in his science and art. In the words of hydraulic engineer and Leonardo scholar Enzo Macagno, “To Leonardo, if not everything, almost everything was flowing or could be in one state of flow or another.”
In early Greek philosophy, the idea that everything in the world is in a process of constant change was expressed in the famous saying by Heraclitus of Ephesus, “Everything flows.” There is no evidence that Leonardo was familiar with the philosophy of Heraclitus, but in an intriguing double portrait by the famous architect Donato Bramante, who was a close friend of Leonardo, Bramante represented his friend as Heraclitus and himself as Democritus.
Since he saw movement and transformation as fundamental characteristics of all natural forms, Leonardo assumed that the basic properties of flow were the same for all fluids, and he found this confirmed by his observations. He emphasized especially the similarity between flows of water and air. “In all cases of motion, there is great conformity between water and air,” he noted in Manuscript A, and in the Codex Atlanticus: “The movement of water within water acts like that of air within air.”
However, Leonardo was well aware that air differs from water in being “infinitely compressible,”
whereas water is incompressible.
As far as flows of liquids were concerned, Leonardo experimented not only with water but also investigated the flows of blood, wine, oil, and even those of grains like sand and seeds. His experiments with granular materials are especially remarkable. He realized that he could learn something about the flow of water by observing a similar but somewhat simpler phenomenon—the flow of grains in which the individual flowing particles are actually visible. This method of using simplified models to analyze the essential features of complex phenomena is an outstanding characteristic of our modern scientific method.
The fact that Leonardo used it repeatedly is truly remarkable. In his view of flow as a universal phenomenon of gases, liquids, and granular materials, and his attempts to use the latter as models of the former, Leonardo’s thought showed a level of scientific abstraction that was centuries ahead of his time.
Modern Fluid Dynamics
In modern science, the field of fluid dynamics (also referred to as “fluid mechanics,” “hydrodynamics,” and “hydraulics”) is notoriously difficult because of the pervasive appearance of turbulent flows that have so far eluded a comprehensive mathematical analysis.
In an oft-quoted phrase, physicist and Nobel laureate Richard Feynman called turbulence “the last unsolved problem of classical physics.”
Turbulent flows are composed of eddies, also known as vortices, in a broad range of sizes, continually forming and breaking down—those swirling and randomly moving patches of fluid that so fascinated Leonardo. The problem in fluid dynamics is that turbulence is not the exception but the rule. At low velocities, the fluid’s internal friction, or viscosity, prevents turbulence from occurring, but as the flow velocity increases turbulence sets in, first at very small scales and eventually spreading throughout the entire fluid.
In the nineteenth century, the mechanical engineer Osborne Reynolds discovered that the onset of turbulence can be characterized in terms of a single parameter, now known as the Reynolds number, which is proportional to the flow velocity, the fluid’s viscosity, and the dimensions of the physical object containing the flow. At low values of the Reynolds number, the flow remains smooth, or laminar, and at a certain critical value it becomes turbulent. This discovery marked a major advance in fluid dynamics. Since different values of the Reynolds number correspond to different types of turbulence, this parameter allows scientists and engineers to compactly characterize turbulent flows.
In spite of this advance, however, scientists have so far not been able to formulate a comprehensive theory of turbulence. The mathematical difficulties arise from the fact that the basic equations of motion governing fluid flow, known as the Navier-Stokes equations, are nonlinear and notoriously difficult to solve. This nonlinearity is the mathematical equivalent of the chaotic nature of turbulence. Any turbulent flow contains a very large number of interrelated variables. The value of any one of these at a particular point depends on the flow at many other points, so that solutions must be obtained at many points simultaneously. This is made even more complicated by the fact that turbulent flows display a broad spectrum of scales. The size of the largest eddies may be over a thousand times that of the smallest, which makes their simultaneous mathematical description exceedingly difficult.
The use of powerful computers to simulate and analyze turbulent flows has recently made it possible to find some solutions for special cases. However, even with the new concepts and techniques of complexity theory, or nonlinear dynamics, scientists and mathematicians have had only limited success. They are able to simulate the onset of turbulence and some simple flow patterns—for example, slow, two-dimensional flows around an obstacle—but full turbulent flows are still frustrating all efforts of comprehensive analysis.
Thus, fluid dynamics today consists of a multitude of theories, each valid only for special cases and each invariably invoking some heuristic hypotheses based on experimental observations. In view of this patchwork of theories and of the tremendous difficulties faced by today’s engineers, physicists, and mathematicians in their attempts to solve the enigmas of turbulent flows, Leonardo’s achievements in this field are truly impressive. At a time long before the development of sophisticated mathematical techniques and powerful computers, Leonardo was able to gain many remarkable insights into the nature of fluid flow.
Leonardo’s voluminous notes on the movements of water remained hidden for several centuries after his death, and hence had no influence on the development of science and engineering. The first theoretical analyses of fluids were undertaken only in the eighteenth century when the great mathematician Leonhard Euler applied the Newtonian laws of motion to an idealized “perfect” fluid (that is, a fluid without viscosity), and the physicist and mathematician Daniel Bernoulli discovered some of the basic energy relations exhibited by liquids. Unlike Leonardo two centuries earlier, however, the hydraulic engineers of that time were not interested in theory, and the theorists did not compare their models with observations of the actual flow of fluids. Real progress in fluid dynamics had to wait until the nineteenth century when Claude-Louis Navier and George Stokes generalized Newton’s equations for the description of the flow of viscous fluids and Reynolds discovered the parameter that now bears his name. It was only then that physicists and mathematicians rediscovered many of the theoretical ideas about fluid motion that Leonardo had clearly formulated more than three hundred years earlier.
Rivers and Tides, Waves and Flows
In his lifelong studies of “the movements of water,” Leonardo observed the flows of rivers and tides, drew beautiful and accurate maps of entire watersheds, and investigated currents in lakes and seas, flows over weirs and waterfalls, the movement of waves, as well as flows through pipes, nozzles, and orifices. A thorough analysis of all his observations, drawings, and theoretical ideas would fill an entire book, and indeed such a book ought to be written. In this chapter I shall concentrate on Leonardo’s discussions of the main characteristics of fluid flow and of turbulence.
Leonardo was well aware of the difference between flow and wave motion. As I discussed at some length in my previous book, he recognized from precise observations of circular ripples in a pond that the water particles do not move along with the wave but merely move up and down as the wave passes by. What is transported along the wave is the disturbance causing the wave phenomenon—the “tremor,” as Leonardo called it—but not any material particles. To make it easier for the eye to follow the precise movements of the water particles, Leonardo threw small pieces of straw into the pond, and he also compared their motion to waves in a wheat field:
The wave flees from the place of its creation without the water changing its position, like the waves which the course of the wind makes in wheat fields in May, when one sees the waves running over the fields without the ears of wheat changing their place.
The comparison between water waves and waves in a wheat field was quite natural for Leonardo, because he saw wave motion as a universal form of propagation of physical effects in all four elements—earth, water, air, and fire (or light). Moreover, he masterfully portrayed the effects of “waves of emotion” in his paintings. This is especially apparent in the ebb and flow of movements in his most grandiose work, The Last Supper.
As several art historians have pointed out, the internal dynamics of the painting can be perceived as a wave movement, emanating from the figure of Christ in the center, spreading outward in both directions, and then being reflected at the end of the table and the edge of the fresco to return once more to the center. A few years before painting the Last Supper, Leonardo had carefully observed interpenetrating waves generated by pebbles thrown into the still water of a pond.
Now he portrayed a similar effect in the realm of human emotions. The words of Christ, “One of you will betray me,” are dropped into the solemn silence of the assembled company, where they generate powerful waves of emotion that propagate and interpenetrate through all the figures in the composition.
During his extensive travels in central Italy, Leonardo often spent time at the seashore, both on the Adriatic and the Ligurian seas. During these visits, he invariably observed and analyzed the nature and types of waves, the breaking of surf upon the shore and the generation of mist, the deposits of debris on the beaches, the ebb and flow of the tides, the impacts of waves on rocky coastlines during sea storms, and other phenomena associated with waves. However, by far the largest part of his work on the movements of water was concerned with the nature of fluid flow.
Leonardo not only observed smooth and turbulent flows of water in rivers and canals and over weirs and waterfalls but also carried out flow experiments in controlled laboratory settings. For example, he would fill vessels of different shapes with water, disturb their surfaces with his hand, and observe the effects. In a simple experiment, he produced a rotational vortex. “When the hand is turned in circular movement in a vase halffilled with water,” he noted, “it generates an artificial eddy that will expose the bottom of this vase to the air.” With the same method, he also generated more complex forms of turbulence: “The hand drawn frequently back and forth across the vase produces strange movements and surfaces of different heights.”
In a series of more sophisticated experiments, Leonardo built special flow channels for observing fine details of water movements. Here is how he described his experimental setup:
Make one side of the channel of glass and the remainder of wood; and let the water that strikes there have millet or fragments of papyrus mixed in it, so that you can better see the course of the water from their movements. And when you have made the experiment of these rebounds, fill the bed with sand mixed with small gravel; then smooth this bed and make the water rebound upon it; and watch where it rises and where it settles down.
These careful and detailed experiments are typical of the empirical method Leonardo used in all his scientific investigations. In his studies of fluid flow, he designed and tested several experimental methods that are still routinely employed in our modern laboratories five hundred years later. Foremost among them were his techniques of flow visualization. Even though he had exceptional powers of observation, he must have realized how difficult it is to accurately perceive the streamlines of turbulent flows. He repeatedly described how he added millet seeds and other types of grains to the water to make it easier for the eye to follow these complex movements:
This experiment you will make with a square glass vessel, keeping your eye at about the center of one of these walls; and in the boiling water with slow movement you may drop a few grains of millet, because by means of the movement of these grains you can quickly know the movement of the water that carries them with it. And from this experiment you will be able to proceed to investigate many beautiful movements.
To analyze turbulent flows in rivers, Leonardo observed the movements of leaves or pieces of wood, and he also used sawdust for making complex streamlines visible:
If you throw sawdust down into a running stream, you will be able to observe where the water, turned upside down after striking against the banks, throws this sawdust back toward the center of the stream, and also the revolutions of the water, and where other water either joins it or separates from it, and many other things.
In other experiments, he used dye to stain one of two coalescing water currents to find out how exactly they merge. These methods of flow visualization were rediscovered in the nineteenth century and have become standard practice in modern fluid dynamics to observe turbulent flows. Leonardo applied similar methods for visualizing the streamlines in flows of air, observing the movement of clouds, snowflakes, smoke, dust, leaves, and other things carried by the currents of the wind. “The air moves like a river,” he explained, “and drags the clouds with it just as running water drags all the things that float upon it.”
On a folio of the Codex Atlanticus, we find a particularly clear and elegant description of flow visualization with millet seeds (fig. 1-2). Leonardo has sketched a glass vessel filled with water and with millet seeds dropping in from above, and he explains that the purpose of the experiment is to find out how exactly the water empties out through a central hole at the bottom. He notes that the seeds drift in from the sides, as the pathlines in the drawing indicate. In another similar experiment, he uses black seeds at the center and white seeds at the periphery in order to see which parts of the water pass through the hole first. It is noteworthy that Isaac Newton drew a diagram similar to Leonardo’s in his famous Principia (Proposition XXXVI, Problem VIII) but got the pathlines wrong because he had no means to visualize them.

FIG. 1-2. Flow visualization with millet seeds. Codex Atlanticus, folio 219r (detail).
The Fundamentals of Flow
After many years of studying and analyzing the movements of water, Leonardo’s sharp observations and methodical experiments gave him a full understanding of the main characteristics of fluid flow. He recognized the two principal forces operating in flowing water—the force of gravity and the fluid’s internal friction, or viscosity—and he correctly described many phenomena generated by their interplay. He also realized that water is incompressible and that, even though it assumes an infinite number of shapes, its volume is always conserved. In addition, he recognized that water changes its properties when materials are suspended or dissolved in it and also when its temperature changes.
In a branch of science that did not even exist before him, Leonardo’s deep insights into the nature of fluid flow must be ranked as a momentous achievement. That he also drew some turbulent structures erroneously and imagined some flow phenomena that do not occur in reality does not diminish his accomplishment, especially in view of the fact that even today scientists and mathematicians encounter considerable difficulties in their attempts to predict and model the complex details of turbulent flows.
Leonardo was the first to study the dynamics of the smooth flow of a river methodically. To do so, he watched corks float down a straight stretch at various distances from the bank and counted “beats of time” to find out how long it took the corks to pass through 100 braccia (approximately 200 feet). He also designed surveying instruments for measuring differences in levels in order to calculate “how much a river falls per mile.” In addition, he used specially designed floats, suspended at varying depths of a flowing river, to determine the water’s relative speeds at different levels.
Leonardo’s analysis of these systematic experiments begins with the recognition that the flow of water is caused by the force of gravity. “One cannot describe the process of the movement of water unless one first defines what gravity is,” he declares, and then proceeds to explain the origin of gravity according to the Aristotelian four-element theory.
According to this view, commonly held in the Middle Ages and the Renaissance, the elements, when left to themselves, settle into concentric spheres with the Earth at the center, surrounded successively by the spheres of water, air, and fire (or light). However, the four elements do not always remain in their assigned realms but are constantly disturbed and pushed into neighboring spheres, whereupon they naturally try to return to their proper places. This is why, according to Aristotle, rain falls downward through the air, while air drifts upward in water, and the flames of fire rise up into the air.
Leonardo’s discussion of gravity in connection with the flow of water clearly follows the Aristotelian theory. When the water is raised out of its sphere, he explains, it acquires weight and is pulled back by gravity, and this is true for all four elements:
All the elements, though they are without weight in their own sphere, possess weight outside their sphere, that is, when moved toward the sky, but not when moved toward the center of the Earth.
For most of his life, Leonardo held on to the Aristotelian concept of gravity as arising from the natural tendencies, or goals, inherent in all matter. But he often struggled when trying to explain various phenomena of mechanics within that traditional teleological framework (see p. 173), and in his mid-fifties, while he was working on organizing his notes on water in the Codex Leicester, he also expressed some doubts about the Aristotelian view:
The water that moves through the river is either called, or chased, or it moves by itself. If it is called, or rather commanded, who commands it? If it is chased, who chases it? If it moves of itself, it shows itself to be endowed with reason, but bodies that continuously change shape cannot possibly have reason, for in such bodies there is no judgment.
However, in his old age Leonardo was much more interested in the growth patterns and metabolic processes in plants, the movements of the human body, and the mystery of the origin of life, than in formulating a theory of mechanics that would go beyond the Aristotelian framework. He never revisited his doubts about the nature of gravity, and it took another two hundred years until the Aristotelian teleological view of forces was replaced by a radically different concept in the physics of Galileo and Newton (see p. 179).
At any rate, for Leonardo’s analysis of fluid flow the Aristotelian view of gravity was sufficient, as it did not contradict any of his observations. When he was in his late forties, he designed a highly ingenious experiment to measure the acceleration due to gravity by using an inclined plane, as Galileo would do more than a hundred years later (see p. 193). Leonardo reasoned that, “although the motion is oblique, it observes in each of its degrees an increase in motion and in velocity in arithmetic progression.” In other words, he asserted that the velocity of a freely falling body is a linear function of time.
Realizing that, in cases of negligible friction, a ball falling freely through the air obeys the same mathematical law as one rolling down an inclined plane, Leonardo then applied the same reasoning to the flow of rivers. “If the rivers were straight with equal breadth, depth, and slant,” he argued, “you would find that with each degree of movement they would acquire degrees of speed.”
It was clear to Leonardo, however, that the analogy between a ball rolling down an inclined plane and water flowing through an inclined riverbed was an idealization that neglected the effects of friction. As an engineer, he paid special attention to friction in his designs of machines and, in fact, was the first to systematically analyze frictional forces (see pp. 185–86). Accordingly, he was keenly aware of the internal friction of fluids, known as viscosity, and dedicated numerous pages in his Notebooks to analyzing its effects on fluid flow.
In modern physics textbooks, viscosity is defined as the internal friction, or “stickiness,” between molecules in a fluid. Except for the concept of molecules, this was exactly how Leonardo described it. “Water has always cohesion in itself,” he wrote in the Codex Leicester, “and this is the more potent as the water is more sticky.” The viscosity of flowing water generates friction between the liquid and its solid container, and also between adjacent layers flowing at different velocities. Leonardo clearly understood the effects of these frictional forces, known in modern fluid dynamics as shear stress. In particular, he recognized that the friction between a solid and a liquid is much larger than that between different layers of the fluid. “The bottom offers more resistance; this is why [the water] moves more on the surface than at the bottom,” he noted in Manuscript H,
and in Manuscript I he recorded that “rivers, when straight, flow with much greater impetus in the center of their breadth than they do at their sides.”
Leonardo’s understanding of the dynamics of the flow of rivers far surpassed that of his contemporaries. Until the second half of the eighteenth century, it was commonly believed that the velocity of water in a river increased from its surface to the bottom. The decrease of the flow velocity near solid walls, such as those of a riverbed, which Leonardo described clearly and accurately in several of his Notebooks, was rediscovered only in the nineteenth century.
The third fundamental characteristic of fluid flow identified by Leonardo (in addition to the effects of gravity and viscosity), was the conservation of mass. He realized that the mass of any portion of flowing water is always conserved, and that, since water is incompressible, this portion will have the same volume no matter what shape it assumes. Leonardo was so impressed with this conclusion that he tried to develop a special type of geometry that would allow him to describe with mathematical precision the continual movements and transformations of water, in which its mass and volume are always conserved. He called this geometry of transformations “geometry done with motion” and worked on it intensely during the last twelve years of his life. Today, we can see that Leonardo’s mathematical transformations were early forms of topology, one of the most important fields in modern mathematics, which was fully developed by Henri Poincaré at the beginning of the twentieth century, some five hundred years after Leonardo’s death.
Leonardo’s explorations of topological transformations never reached a stage where they could actually be used to model fluid flows. However, even though his mathematical techniques were not sophisticated enough for this ambitious task, he seems to have been on the right track. Instead of his topological transformations of visible geometric forms, physicists today use a mathematical method known as tensor calculus to describe continuous transformations of infinitely small volumes of fluids under the influence of gravity and shear stresses. The conservation of mass, expressed in the so-called continuity equation, is a cornerstone of the modern mathematical theory, as it was for Leonardo.

FIG. 1-3. Streamlines of a breaking wave. Ms. F, folio 20r (detail).
Realizing that the mathematics of his time was inappropriate for describing the ceaseless movements and transformations of flowing water, and that his own geometry of transformations was too rudimentary for modeling complex flow phenomena, Leonardo chose a third option. Instead of mathematics, he used his exceptional facility of drawing to document his observations in pictures that can be strikingly beautiful while at the same time playing the role of mathematical diagrams. His numerous drawings of turbulent flow patterns are not realistic representations of single instances of observation, but are syntheses of many observations in the form of theoretical models. They range from simple wave patterns, in which the individual streamlines are labeled with letters (fig. 1-3), to highly complex combinations of swirling eddies and bubbles, as in the drawing of “water falling upon water” in the Windsor Collection (see fig. 1-13).
Measuring the Flow of Water
During the late fifteenth century, when Leonardo was employed as ducal engineer at the Sforza court in Milan and had to oversee numerous projects of hydraulic engineering as part of his duties (see p. 32), the measurement of the flow of water was an important technical and administrative issue. Agriculture in the expansive Lombard plain depended crucially on effective irrigation through the region’s large network of rivers and canals, and all administrative and legal questions connected with water rights, concessions, and payments required accurate measurements of rates of flow under varying conditions.
However, the unit of measurement for the rate of flow (the volume flowing through a given cross section per unit of time) that was commonly used in Lombardy was based on an erroneous assumption, which made a fair and equitable distribution of water virtually impossible. Known as the oncia milanese (“Milanese ounce”) this measure had been in use since the twelfth century. It was defined as the quantity of water discharged by a rectangular orifice of a certain dimension (equivalent to approximately 6” × 8”). While it is evident to us that the actual rate of flow also depends on the velocity of the water discharged by the orifice, which in turn depends on the level of water upstream, rates of flow were measured for hundreds of years simply in terms of cross-section areas without taking velocity into account.
Leonardo’s discussions of measuring and calculating quantities of flow, by contrast, exhibit a conceptual clarity that is truly impressive. It is a clarity derived from his thorough understanding of the fundamental characteristics of fluid flow and from his considerable powers of visualization. He realized that the rate of flow through a given cross section must be defined as the volume flowing through that area per unit of time and that, since the flow velocity is defined as the distance traveled by the water per unit of time, the rate of flow equals the product of cross-section area times flow velocity. The fact that the rate of flow is proportional not only to the area but also to the flow velocity is clearly stated in several Notebooks. In Manuscript F, for example, Leonardo writes:
The quantities of ounces of water delivered through an orifice will be greater or lesser according to the higher or lower velocity of the water. Doubling the velocity will double the water in the same time; and in the same way, tripling the velocity will triple the quantity of water in the same time; and so it would go on to infinity.
In fact, Leonardo was not the first to understand the concept of the rate of flow. The Greek engineer Heron of Alexandria had done so already in the first century A.D. In his Dioptra, a book on land surveying, Heron discussed the measurement of the flow of water from springs, and in this context he correctly defined the rate of flow and also stated the precise relationship between rate of flow, area, and velocity. However, Heron’s work was subsequently forgotten and remained unnoticed for almost fifteen hundred years, until his insights were rediscovered by Leonardo at the end of the fifteenth century.
When he studied the flow of rivers, Leonardo correctly conceived of the rate of flow as the volume flowing through a particular cross section in a “beat of time,” and since he knew that volume is conserved in all flows, he concluded that under steady flow conditions, “the river transports in every section of its length in the same time the same quantity of water.” He also realized that this implies that in steady flows, cross-sectional areas and flow velocities are inversely proportional:
A river of uniform depth will have a more rapid flow at the narrower width than at the greater, to the extent that the greater width surpasses the narrower.
This is a clear and succinct statement of an important principle of fluid dynamics, known today as the continuity principle. It is the mathematical expression of the fact that in a steadily flowing river, the flow needs to speed up in narrower passages to accommodate the same quantity of water. To illustrate this flow dynamic, Leonardo used the ingenious analogy of a regiment marching through a street of varying width while keeping its compact formation. A sketch in Manuscript A schematically shows a street with men marching through three sections with widths widening progressively in the ratios 1 to 4 to 8. In the accompanying text, Leonardo explains:
Suppose that the men fill these avenues with their bodies, and that they must march in a continuous manner. When the men in the widest place take one step, … those in the intermediate width will take 2, and those in the third place, with a width one fourth of the second, will take 8 steps during that same time. And you will find this proportion in all movements that pass through places of varying widths.
In representing the flow of water in a river of varying cross section by a regiment of men marching through a street of varying width, Leonardo used a simplified, or approximate, model to analyze the essential features of the phenomenon he studied. In subsequent centuries, this technique became a central feature of the modern scientific method. Most advances in science today are presented first in terms of such approximate models and are cast into more comprehensive and elaborate theories only much later. That Leonardo already used this modern scientific approach in the fifteenth century is quite extraordinary.
Leonardo’s precise formulations of the rate of flow and the principle of continuity remained unpublished until the nineteenth century and had no influence on hydraulic engineering in his time, so far as we know. Rates of flow continued to be measured in terms of the flawed unit of the oncia milanese for another three hundred years, and the continuity principle, although known to hydraulic engineers at least qualitatively, was not formulated precisely again until Benedetto Castelli, a pupil of Galileo, did so in the seventeenth century. Thus, until the middle of the nineteenth century, Castelli rather than Leonardo da Vinci was considered the founder of the Italian school of hydraulics.
The Water Vortex
At the center of Leonardo’s investigations of turbulent flows lies the water vortex, or whirlpool. Throughout the Notebooks, there are countless drawings of eddies and whirlpools of all sizes and types. These often very beautiful drawings are testimony to Leonardo’s endless fascination with the ever-changing yet stable nature of this fundamental type of turbulence. As I have suggested earlier, this fascination likely came from a deep and correct intuition that the dynamics of vortices, which combine stability and change, embody an essential characteristic of all living forms (see p. 22).
Leonardo described correctly how eddies are formed when flowing water encounters an obstacle, such as stagnant water. “The current of moving water seeks to maintain its course according to the force that caused it,” he wrote in Manuscript A, “and when it finds an opposing obstacle, it completes the length of the course it began by circular and whirling movements.” On the verso of the same folio, Leonardo illustrated this process with a sketch of water flowing into a tank (fig. 1-4). His observation that the circular flow will run its course until the energy is dissipated, just as it would if it moved in a straight line, is completely correct. The drawing itself is partly incorrect, however, as in reality only one pair of counter-rotating vortices is formed.

FIG. 1-4. Formation of counter-rotating vortices by water flowing into a tank. Ms. A, folio 60r (detail).
Leonardo was the first to study and understand the detailed motions of water vortices, often drawing them accurately even in complex situations, for example in wakes behind boats and other obstacles in turbulent water. He correctly distinguished between flat circular eddies in which the water essentially rotates as a solid body, and spiral vortices (such as the whirlpool in a bathtub) that form a hollow space, or funnel, at their center. “The spiral or whirling motion of every liquid,” he noted, “is so much swifter as it is nearer to the center of its revolution. This fact, which we point out, is worth noting, since the circular motion of a wheel is so much slower as it is nearer to the center of the revolving object.”
In fact, circular eddies are not exactly flat but dip slightly in their center. As the rotational velocity increases, this dip becomes more pronounced and the circular eddy turns into a spiral vortex. Leonardo observed accurately that the hollow space at the center of such a vortex will deepen with increasing rotational velocity:
The eddy with the deeper hollow will be the one produced in water of swifter movement; and that eddy will have a smaller hollow if it is produced in deeper water which has not the same movement but is slower.
Based on these observations, Leonardo identified three types of eddies: those level with the surface, those with raised centers (for example, in air turbulence), and those with depressed centers (for example, the whirlpool in a bathtub). He described the three types as “leveling the bottom,” “filling up the bottom,” and “hollowing out the bottom,” respectively. Leonardo’s detailed studies of vortices in turbulent water and air were not taken up again for another 350 years, when the physicist Hermann von Helmholtz developed a mathematical analysis of vortex motion in the mid-nineteenth century.
For many years, Leonardo observed whirlpools and vortices in the currents of rivers and lakes, around piers and jetties, at the confluence of rivers, in the basins of waterfalls, behind objects of various shapes immersed in flowing water, and in laboratory tanks. He repeatedly attempted to classify different types of vortices according to their shapes—their topology, as we would say today. He made long lists of these types of turbulence. Here is a typical example from Manuscript F:
Of the eddies on the surface and of those created at various depths of the water; of those that take up the whole of that depth, and of the moving and the stable ones; of the long and the round ones; of those that change their movement periodically and those which divide; of those which convert into the eddies they merge with; and of those that are mixed with falling and reflecting water and make it spin around.
What kind of eddies turn light objects on the surface without submerging them? What kind are those that submerge them and make them spin around at the bottom, and then leave them there? What kind are those that detach things from the bottom and throw them up to the surface of the water? Which are the slanting eddies, which are the upright ones, and which the level ones?
If this list sounds confusing, it will be worth pointing out that even today, five hundred years after Leonardo, there is still no commonly accepted classification scheme for different types of eddies. As fluid dynamicist Ugo Piomelli observes wryly: “If you ask 200 turbulence experts, you will get between 190 and 200 different answers. And, what is more interesting, you will even find 10 or 20 different definitions of what an eddy is.” In other words, Leonardo’s seemingly confusing classification schemes reflect, more than anything else, the great dynamic complexity of those swirling, ever-changing vortices.
Turbulent Flows
Looking through page after page of Leonardo’s depictions and descriptions of turbulent flows, one is astonished to see how many subtle and complex features of turbulence he identified that are confirmed in modern fluid dynamics. For example, as noted earlier, turbulent flows often display a broad spectrum of scales with eddies of many different sizes forming continually (see p. 34). Leonardo was well aware of this fact:
The great revolutions of eddies are rare in the currents of the rivers, and the small eddies are almost innumerable; and large objects are turned around only by large eddies and not by small ones, whereas the small objects are made to spin both by small eddies and by large ones.
Leonardo recognizes here not only a whole spectrum of eddies of different sizes, but also a corresponding range of angular momenta, illustrated with objects of different sizes carried by the eddies.
The Windsor Collection contains two beautiful drawings of a jet of water falling into a pool (figs. 1-5 and 1-13). In figure 1-5, Leonardo depicts the formation of a broad range of turbulent structures of various sizes. The region of turbulence is divided into two parts: a “frozen” regime showing different types of stable vortices, and a regime drawn in lighter chalk, in which the turbulent kinetic energy cascades down through vortices of smaller and smaller scales until it dissipates in the water’s viscosity. (The small sketch above the main drawing summarizes the principal movement of falling and reflected water, while the sketch on the left is a visual reminder of the connection between circular waves and wave fronts.)

FIG. 1-5. Study of turbulence generated by a jet of water falling into a pond, c. 1509–11. Windsor Collection, Landscapes, Plants, and Water Studies, folio 44 (detail).
The entire drawing is an elaborate diagram of Leonardo’s understanding of turbulence generated by the jet of water, which is shown to be correct in its essential aspects in modern flow visualizations. Its most remarkable feature is the energy cascade through vortices of decreasing scales and subsequent energy dissipation, known as a Richardson cascade in modern fluid dynamics, which Leonardo qualitatively anticipated five hundred years before it was formulated by the physicist Lewis Richardson.
A large number of Leonardo’s studies of turbulence are concerned with the flow of water around immersed objects, known in modern fluid dynamics as bluff body flows. In the small Notebook known as Manuscript H, for example, which Leonardo carried with him during his observations of flowing water in nature, we find a series of sketches of bluff body flows around objects of various shapes (fig. 1-6). As in many other similar drawings, Leonardo here correctly shows how the flow pattern is attached to the object upstream but generates separated vortices (so-called vortical shedding) downstream.

FIG. 1-6. Flow patterns around immersed objects, c. 1493–94. Ms. H, folio 64r.
When looking at these sketches, it is important to realize that they are not realistic, instantaneous pictures of actual flow lines. Leonardo probably observed a series of fluctuating flow patterns, visualizing them with the help of a sequence of millet seeds, identified certain types of turbulence, and then recorded his observations in summary diagrams. Hence, the sketches we see in the Notebooks are superpositions of observed instantaneous structures, resulting in pictures of average flow patterns. They are Leonardo’s conceptual models of turbulent flows.
For example, the vortex pairs in the top two sketches in figure 1-6 would not appear simultaneously but alternately in rapidly changing, fluctuating flows. What the sketches show are the average flow patterns. The same technique is used by scientists today with the help of sophisticated time-lapse photography. Centuries earlier, Leonardo relied on simple methods of flow visualization and on his acute powers of observation to achieve remarkably similar results.

FIG. 1-7. Bluff body flow around a column. Codex Leicester, folio 22r (detail).
The Codex Leicester is full of sketches of bluff body flows, neatly arranged along the right-hand margin of the text. Figure 1-7, for example, shows water flowing around a column. Again, Leonardo has drawn a symmetrical picture recording the average flow pattern. The actual flow would consist of staggered fluctuating vortices. Similarly, the counter-rotating pairs of vortices in figure 1-4 should be interpreted as symmetrical averages of the actual flow pattern.
In another remarkable drawing, also from the Codex Leicester, Leonardo correctly depicts what is now called a horseshoe vortex around a prism-shaped obstacle (fig. 1-8). The basic shapes of the head and the two legs of the horseshoe are clearly shown. However, the direction of rotation of the head is incorrect. It should be clockwise, not counterclockwise as drawn by Leonardo. (Since the water on the surface flows faster than that below, the top layer will turn down and then rise up again when the flow encounters the obstacle.)

FIG. 1-8. Horseshoe vortex. Codex Leicester, folio 25v (detail).
In addition to the relatively simple sketches in the Codex Leicester, Leonardo produced several elaborate drawings of highly complex patterns of turbulence, generated by placing various obstacles into flowing water. Figure 1-9, from the Windsor Collection, shows the turbulent flows around a rectangular plank inserted at two different angles. (Additional variations are suggested in the small sketches to the right of the main drawing.) The upper drawing clearly shows a pair of counter-rotating vortices (fluctuating in the actual flow) at the head of a stream of random wake. The essential details of this complex pattern of turbulence are completely accurate—a testimony to Leonardo’s powers of observation and conceptual clarity.

FIG. 1-9. Turbulent wakes behind a rectangular plank, c. 1509–11. Windsor Collection, Landscapes, Plants, and Water Studies, folio 42v (detail).
As noted earlier, the origins of modern fluid dynamics go back to the nineteenth century, when Osborne Reynolds identified a parameter that allowed scientists and engineers to neatly characterize different types of turbulence (see p. 34). At the same time, Reynolds also discovered that the mathematical description of turbulent flows can be decomposed into a main flow (averaged over time) and a fluctuating part. Leonardo also recognized the difference between these two components of turbulent flows, albeit only in a qualitative way. On a famous sheet of the Windsor Collection, he compared the turbulent flow of water to the growth of curly hair. He drew three turbulent wakes behind rectangular obstacles next to a drawing of a curly lock of hair that looks virtually identical (fig. 1-10). Below the drawings, he noted: “Observe the motion of the surface of the water, which resembles that of hair, which has two motions, one of which is caused by the weight of the hair, the other by the direction of the curls.” And then he continued:

FIG. 1-10. Turbulent wakes and strands of curly hair, c. 1510–13. Windsor Collection, Landscapes, Plants, and Water Studies, folio 48r.
Thus, the water has its eddying motions, one part of which is due to the impetus of the principal current, the other to the incident and reflected motion.
With this statement, Leonardo qualitatively anticipated what is now known as the Reynolds turbulence decomposition nearly four hundred years before Osborne Reynolds formulated it mathematically.
In all his observations of turbulent flows, Leonardo clearly recognized that, in spite of incessant changes and fluctuations, turbulence also produces surprisingly stable forms. With this recognition he anticipated another important concept of modern fluid dynamics, where these organized forms of turbulence—whirlpools and vortices at all scales—are known as coherent structures. Leonardo drew them repeatedly, and he commented on their stability in several Notebooks. In Manuscript I, for example, we find the following rather precise observation:
Eddies, with various revolving movements, proceed to consume the initial impetus; and they do not remain in the same positions, but after they have been generated, turning thus, they are borne by the impetus of the water in the same shape, so that they come to make two movements: one they make within themselves by their revolution; the other as they follow the course of the water that carries them along.
Like so many of Leonardo’s acute observations on turbulence, this one, too, is fully borne out by modern flow visualizations.
Water Falling upon Water
A type of turbulent pattern that Leonardo found particularly intriguing is that caused by a jet of water falling into a calm pool. Manuscripts A, F, and I, as well as the drawings in the Windsor Collection, contain many studies of waterfalls and jets impinging on still water. In several of them, the jet enters the area above the pool through a rectangular orifice, an “industrial” feature that is reminiscent of Leonardo’s frequent work on locks and canals.
In some of these studies, Leonardo draws turbulent structures of a special kind, which have been called “corkscrew waves” by art historians (fig. 1-11). These helical structures do indeed occur in special types of turbulence. I believe, however, that what we are seeing in Leonardo’s sketches are not records of actual observations, but rather a particular theoretical model that he uses to explain the vortices generated in waterfalls. Indeed, in the Codex Atlanticus, there is an elaborate drawing of a rope coiled first into a helix and then into a double helix (fig. 1-12), which is reminiscent of the helically coiled vortices in the waterfall drawings. This strongly suggests that Leonardo used such a rope as a model for his torus- (or “doughnut”-) shaped vortices.

FIG. 1-11. Waterfall and “corkscrew waves.” Windsor Collection, Landscapes, Plants, and Water Studies, folio 45r (detail).

FIG. 1-12. Rope coiled into a helix and a double helix. Codex Atlanticus, folio 520r (detail).
Leonardo’s most elaborate studies of water falling into a pool are the two drawings in the Windsor Collection mentioned earlier (see p. 49). His celebrated drawing of “water falling upon water,” in particular, is an impressive synthesis of numerous previous studies, which integrates various types of turbulence into a compact and beautiful picture (fig. 1-13). It is obvious that this is not a realistic snapshot of a jet of water falling into a pond, but an elaborate diagram of Leonardo’s analysis of the complex set of turbulences caused by the jet.

FIG. 1-13. “Water falling upon water,” c. 1508–9. Windsor Collection, Landscapes, Plants, and Water Studies, folio 42r (detail).
The accompanying description begins with the statement that there are four types of turbulence involved:
The movements of the falling water after it has entered the pool are three in kind, and to these a fourth is added, which is that of the air submerging itself with the water.
Leonardo then continues with a detailed description of these movements, which goes over several paragraphs and is rather confusing. The four movements he has identified seem to be the movement of water and trapped air vertically down into the pool; the return of water and air bubbles up to the surface; the bursting of air bubbles and their water falling back into the pool; and the swirling movement of water on the surface in the form of large eddies.
Leonardo’s analysis is a mixture of accurate observations and erroneous assumptions. He correctly observes that air is drawn into the water (a process known today as “entrainment”), and that the water bubbles rise to the surface where they burst into clusters of rosettes. However, he erroneously assumes that after the bubbles burst, their water not only falls back onto the surface but penetrates the pool down to the bottom. In the drawing, this strange assumption is illustrated with a series of oval, downward slanting eddies. The fourth type of turbulence, finally—the large eddies generated on the periphery around the jet’s impact—is again an illustration of a correct observation. Modern flow visualizations indeed show that the eddies become progressively larger as they move away from the zone of impingement.
In a striking contrast between his written and visual analysis, Leonardo’s verbal analysis is tortuous and clumsy, while his visual analysis is compact and beautiful. Both are partly incorrect, but Leonardo was able to take a very chaotic situation and simplify it in terms of a drawing that serves as a concise mathematical diagram. This is exactly what chaos theorists do today. They bring order into chaos in terms of visual lines and shapes, albeit in an abstract mathematical space.
Turbulence in Water and Air
As noted earlier, Leonardo studied not only the flow of water but also the flows of blood, wine, oil, and even flows of various grains. He recognized that the basic properties of flow are the same for all fluids, and he emphasized especially the similarities between flows of water and air (see p. 33).
The currents and turbulences of air were of special interest to him because an understanding of them was crucial to the pursuit of one of his great passions—the science of flight and the design of flying machines (see pp. 250ff.). “In order to give the true science of the movement of birds in the air,” he declared, “it is necessary first to give the science of the winds.” To develop such a science of the winds, Leonardo observed and analyzed the swirling movements of air as meticulously and persistently as he studied the turbulences in flowing water.
In addition, Leonardo frequently studied turbulences in mixtures of water and air. Throughout his life, he was fascinated by natural catastrophes, and he produced numerous drawings of floods and tempests full of swirling currents of air, mixed with rain and pieces of uprooted trees, sand, and rocks. These studies culminated in a series of a dozen extraordinary drawings in somber black chalk known as the “deluge drawings,” which are now part of the Windsor Collection and are accompanied by powerful narratives of apocalyptic visions. Leonardo created them in Rome at the age of sixty-two when he was lonely, depressed, and given to morbid thoughts.
The deluge drawings are violent and disturbing. They show trees being uprooted and torn to pieces, rocks crumbling in the torrential rains, houses and entire towns being demolished by raging storms. The air is dark and gloomy. The landscape can just barely be made out. There are no human victims to be seen; they are rendered irrelevant by the huge scale of the deluge. The overwhelming impression is one of despair, of human frailty and futility in the face of nature’s cataclysmic forces.
The first drawing in the series (fig. 1-14) shows the beginning of the deluge. In the upper right corner, clouds gather ominously and the first swirling vortices of rain-soaked air have formed. In the landscape below, trees are bent down to the ground and water in a lake is stirred up into huge waves by the violent storm. In a further drawing (fig. 1-15), we see the deluge in full force. The sky is filled with with giant menacing vortices; torrential rain is falling; a town is being devastated by the cyclone; rocks are whirled through the air by powerful eddying currents.

FIG. 1-14. The beginning of the deluge, c. 1514. Windsor Collection, Landscapes, Plants, and Water Studies, folio 57.

FIG. 1-15. Cyclone overcoming a town, c. 1514. Windsor Collection, Landscapes, Plants, and Water Studies, folio 62.
Figure 1-16 shows the same raging vortices of water, air, and crumbling rocks, but here Leonardo has formalized his representation of the cataclysm to such an extent that the emotional impact is diminished and the drawing is much closer to a mathematical diagram. In the accompanying narrative, accordingly, highly emotional passages are interspersed with detached, analytical ones that include precise descriptions of cascades and currents and detailed instructions on how to paint optical effects generated by storm clouds and falling rain.
These drawings are superb examples of Leonardo’s synthesis of art and science. He drew them from memory in his old age, not to present the results of observations or experiments, but to picture a mythical situation—the great deluge, the ultimate turbulence. The drawings carry a powerful emotional message, and at the same time they are astonishingly accurate in their renderings of cascades and currents in water and air.
Looking through the entire set of drawings, one can recognize many of the shapes Leonardo explored in his lifelong observations of turbulent flows. There are two-dimensional eddies (for example, in the upper left corner of fig. 1-16); three-dimensional spirals (top of fig. 1-15); torus-shaped vortices (upper part of fig. 1-14); pairs of counter-rotating eddies (upper part of fig. 1-16); and “corkscrew waves,” created by the impact of rain on the ground (right side of fig. 1-16). An archetypal, idealized vortex is shown again and again in different scales and from different perspectives. Taken together, these deluge drawings are nothing less than a visual catalogue of cascades and vortices. They are Leonardo’s final summing-up of his knowledge of turbulence.

FIG. 1-16. Formalized deluge study, c. 1514. Windsor Collection, Landscapes, Plants, and Water Studies, folio 59.
The Spiral Form
In most of the deluge drawings, as well as in the study of “water falling upon water” (see fig. 1-13), Leonardo drew large eddies with small spirals at their ends. These forms are inaccurate. In reality, the turbulences would be circular or elliptical, but the differences can be very subtle and difficult to detect without sophisticated experimental techniques.
I believe that Leonardo liked to draw these eddies with spirals at their ends because he saw the spiral as an archetypal form of turbulent flow and, more generally, as a symbol of life. I have argued earlier that he intuitively recognized the dynamic of the spiral vortex—stable and yet continually changing—as symbolic of all living forms (see p. 22), and I believe that this can also be said of the spiral form in general. In his wide-ranging observations of natural forms, Leonardo could not fail to notice the spiral growth patterns of marine shells and plants. He paid special attention to the ways in which leaves or branches spiral around a central axis in many species of plants and trees, and he drew these spiral growth patterns with complete botanical accuracy (see p. 106). Sometimes, Leonardo also drew exaggerated forms of spiraling foliage, the most famous being that of the “Star of Bethlehem” (plate 6), which bears a striking resemblance to a water vortex.
Leonardo’s fascination with spiral movements can also be seen in many of his paintings, especially in the portraits. With his frequent use of spiral body configurations (for example, in the Saint John the Baptist and the Leda), Leonardo created the form of the serpentine figure that was used extensively by Michelangelo and became one of the fundamental forms of elegance in the High Renaissance. In the words of art historian Daniel Arasse: “Leonardo used the serpentine form to bring life and movement into his figures, thus inventing the classic ‘gracefulness’ of representation.”
For Leonardo, the spiral form was the archetypal code for the ever-changing yet stable nature of living forms. He saw it in the growth patterns of plants and animals, in curling locks, in human movements and gestures, and above all in the swirling vortices of water and air. The movement of water is the grand unifying theme in Leonardo’s science of living forms. Water is the life-giving element flowing through the veins of the Earth and the blood vessels of the human body. It nourishes and sustains all living bodies. Its forms, like theirs, are fluid and always varying. It is a major source of power and for eons has shaped the surface of the living Earth, gradually turning arid rocks into fertile soil. With its infinite variety of form and movement—as rivers and tides, clouds and rain, cascades and currents, eddies and whirlpools—water flows through Leonardo’s art and interlinks the main fields of his science.