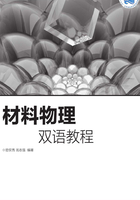
1.3 Brief History of Material Development
Materials played the key role in the history of world civilization and the progress of humankind development. The most important aspect of materials is that they are enabling; materials make things happen. For example, in the history of civilization, natural materials used, such as stone, iron, and bronze, shown an important milestone in mankind's development, even coined the names of historical periods, such as Stone Age, Chalcolithic Age, Bronze Age and Iron Age. In today's fast-paced world, the discovery of making high quality silicon single crystals and the understanding of their electricity properties open the door of the information age.
In many cases different cultures leave their materials as the only records which anthropologists can use to define the existence of such cultures. The progressive use of more sophisticated materials allows archeologists to characterize and distinguish between peoples. This is partially due to the major material of use in a culture and to its associated benefits and drawbacks. For example, the innovation of smelting and casting metals in the Bronze Age started to change the way that cultures developed and interacted with each other. Starting around 5500 BCE, early smiths began to re-shape native metals of copper and gold - without the use of fire - for tools and weapons. The heating of copper and its shaping with hammers began around 5000 BCE. Melting and casting started around 4000 BCE. Metallurgy had its dawn with the reduction of copper from its ore around 3500 BCE. The first alloy, bronze came into use around 3000 BCE. Iron-working came into prominence from about 1200 BCE.
Prehistorically, before the consummation of material science, human beings had learned to use materials by empirical knowledge, the materials include: (1) organic materials: wood, ivory, bone, fiber, and rubber;(2) inorganic materials: minerals and ceramics including stone, flint, mica, quartz, clay, and diamond; (3) metals and alloys: iron, copper, silver and gold.
Just as shown in Figure 1.1, the accumulation of empirical knowledge pushed human civilization forward, which renders the creation of scientific knowledge that further promote the civilization and give insight to empirical knowledge. Thus, the science and technology on materials were gradually developed, which can further promote the development of new materials and explore their unique applications.

Figure 1.1 Both the empirical and scientific knowledge promote the progress of human civilization
Historically, in the development of material science, the discovery of modern atomic and molecular theory is the fundamental basis of all materials science and a key to understanding material structure and processes, which includes the following milestones:
In 1869, Dmitri Mendeleev published the periodic table of the elements, correlating all atoms in materials.
In 1900, Planck's hypothesis well explained the blackbody radiation by introducing the concept of quantum for radiation.
In 1901, X-ray was found by the German physicist W. K. Roentgen. Since then, the X-ray analysis technology has been used in material science to investigate the construction and structure of materials.
In 1905, Einstein used photon theory explained the photoelectric effect, evidencing the particle nature of light.
In 1911, super conductivity was found by Kamerlingh Onnes in mercury at temperature of 4.2 K, adding a new special member in conducting materials.
In same year 1911, Rutherford introduced his 'classical' atomic model consisting of small nucleus surrounded by electrons, firstly disclosing the core-shell structure of atoms.
In 1924, de Broglie suggested wave particle duality, initializing an approach of quantum dynamic explanation between material macro-properties and microstructure.
In 1928, Bloch's theorem explained the conductivity of metal, which was further developed by Wilson in 1931 to insulator.
In 1960, the practical development of field effect transistor leads to the development of integrated circuit (IC).
In 1960, the first laser (ruby laser) was invented.
It can be said that the science of material began with metals, which was termed as metallurgy. Metals possess excellent mechanical properties such as formability and strength, thus serve as prime materials for structural applications. Additionally, their excellent electrical and thermal conductivity has rendered them indispensable for electrical engineering. Despite its very long tradition, metallurgy is not a classical scientific discipline itself. Up to medieval ages, the knowledge of the extraction and fabrication of metals had been considered as a national secret asset and had been only traded orally from generation to generation.
As a matter of fact, metallurgy was a discipline of alchemies in medieval times and comprised a colorful mixture of empirical recipes and superstition. With increasing scientific character of more recent centuries metallurgy became a discipline of chemistry, where it remained even up to now at many universities. The rapid development of the technology to understand the material properties, in particular due to the discovery of radiative beam, such as X-rays, electron beam and ion beam, and their application to material composition and structure, revealed that the properties of metals were not determined by the gross chemical composition, in contrast to common belief at that time. This made metallurgy become a discipline of physical chemistry.
The development of the atomistic foundations of our understanding of mechanical and electronic properties of metallic materials in the frame work of dislocation theory and electron theory of metals finally shifted the focus of metallurgy to physics at the beginning of the 20th century. Eventually it engendered the discipline of metal physics, which has dominantly influenced the science of metals in the past 50 years. In fact, our current understanding of metallic and non-metallic materials on the basis of atomistic models has essentially been developed only in the past 80 years of research in metal physics. The objective of this research has been to describe the properties of a material on the basis of atomistic physical models, which can be formulated in terms of equations of state. This allows for a prediction of materials behavior on a theoretical basis and changes the material research from try and error to the theoretical guiding. This will significantly reduce the cost and time on the experimental investigations and testing of materials behavior.
In the sixties and seventies of the 20th century it became obvious that the urgent demand for high performance materials and competitive mass products had to include the development of non-metallic materials, for instance ceramics for high temperature components and plastics for a weight savings in automobiles and airplanes. Materials research revealed soon, however, that the foundations of physical metallurgy within limits can be readily applied to other materials, in particular to crystalline solids. Crystallography, constitution, diffusion, phase transformations, physical properties, and so on, are the foundations of the understanding of all kinds of engineering materials. Of course, there are also specific differences. For instance, dislocation theory, which is indispensable for an understanding of plastic deformation of metals, is of less importance for brittle ceramics, but it teaches the reasons for the brittleness and, therefore, offers respective for counter actions. For polymers which are usually non-crystalline, an appropriate dislocation concept of their mechanical properties is still too complicated to be useful, and the deformation behavior of plastics is, therefore, currently restricted to phenomenological tribological models.
Material development generated the strong belief that it is possible to derive a comprehensive description comprising the different classes of materials and that the future world be multi-material. This worldwide trend in the seventies of the past century caused the classical independent disciplines of metallurgy, ceramics, and plastics to merge to a new discipline "material science and engineering", encompassing both the science and engineering aspects of materials, and which has become our modern powerhouse of materials research and development.